Department Head Musings: Safety and Aerospace Autonomy
While maintaining our traditional strengths across aerospace engineering, our department has also grown substantially into the area of aerospace autonomy over the last two years. Autonomy has exploded in the capabilities it brings to the aerospace industry. In some cases, ‘autonomy’ reflects the increasing capability for machines to take over functions traditionally performed by humans. This definition of autonomy as a machine, relative to a human, reflects the tension between them. We already have substantial automated capabilities in the safest system ever — air transport — but this domain is finding that this automation, while preventing some failure modes, also creates new ones.
Indeed, the complexity of the autonomous capabilities is frequently a contributor to recent accidents; human pilots remain the ultimate backup, and to keep them primed and current, recent FAA Safety Alerts for Operators request airlines to have their pilots manually fly more as their flight deck becomes more automated. (For a recent discussion of safety and automation in air transport aircraft, search YouTube for the ALPA forum on Automation Exceptions, a panel this past year discussing? I was one of the speakers, so there is a Penn State connection there.)
Ethical Implications
These paradoxical tensions between human and machine raise ethical implications. Alan Wagner, an assistant professor on our faculty since 2016 who was recently recognized with a Hartz Family Career Development Professorship in Engineering, is examining these ethical tensions collaboratively with the Penn State Rock Ethics Institute. At its most basic, he is examining how humans interact with machines that might lie or fail at their tasks—or how one would respond in a game of Connect4 with his robot ‘Baxter’ if it cheats.
His work extends specifically into aerospace applications of autonomy, examining how we help humans understand how much they should ‘trust’ the machine, which is a multifaceted question including whether the human can understand what the machine is doing, believe it is correct, and decide to rely on it—and what the machine should do in support of this interaction.
Questions of Trust
Questions of trust can also be framed theoretically, i.e., how much are the machine’s functions trustworthy? Autonomous functions depend on input information which is uncertain—and then aerospace autonomy operates in an environment which is variable and prone to disturbances and faults. So, the judgments, decisions, and control actions made by autonomy reflect this variability.
The theory of how uncertainty propagates through autonomous functions is the focus of another recent hire, Associate Professor Puneet Singla, who joined our faculty in August 2017. His applications span both space, including studies of space situation awareness and statistical orbit determination, and aircraft, such as identifying the best of UAS as sensing platforms to collectively assess the ‘best’ estimate of the spatio-temporal phenomenon of interest. For example, tracking of toxic material plumes subsequent to natural or man-made disasters such as the Eyjafjallajo ̈kull eruption and the Deepwater Horizon oil-spill, and geomagnetic survey for GEOINT application.
Gaining Control
‘Autonomy’ also spans cases where machines perform functions that humans can’t do, and thus, expands our vision of what aerospace operations are possible. Professor Joe Horn, for example, is examining helicopter landings on small ships where heavy seas and the turbulent wake of the wind around the ship’s super-structure make landings difficult and dangerous. His new flight simulator is coming together in the basement of Hammond Building, with a wide field of view visuals, motion base, and control loading.
Notably, through additional work provided by Associate Professor Sven Schmitz, the simulator will be joined with high-powered computational fluid dynamic analysis such that, as pilots try to land on the ship, the simulator can portray how the dynamics they command of their rotorcraft will interact with the vehicle wake.
Ultimately, Professor Horn seeks to make autonomous flight control such that pilots’ control inputs into the vehicle can allow them to simply land the vehicle despite the complexities in the vehicle dynamics and aerodynamics.
Exploring New Worlds
Further expanding our vision of what is possible, our Dragonfly team, led by Associate Professor Jack Langelaan, and including Schmitz and Assistant Professor Jose Palacios, is taking autonomy to Titan, Saturn’s largest moon. The rotor system is being tested in Palacios’s Adverse Environment Rotor Test Stand in cryogenic conditions.
In a single flight, Dragonfly can explore more of another world than the combined distance traveled by the Mars rovers. At its great distance from Earth, it will need to not just fly itself but manage itself—exploration enabled by autonomy.
Expanding What’s Possible
Professor Eric Johnson, who joined our faculty in August 2017, is setting up the Penn State UAS Research Lab (PURL) and is also developing autonomous vehicles that defy simple classifications. Working with the U.S. Naval Research Laboratory, he and his research team are developing ‘Cormorant’ quadcopters that can land in water, take on water to attain neutral buoyancy, and then ‘fly’ underwater. These developments are exciting for how they are using autonomy to expand on what operations are possible.
Adapting to the Times
Further, we are positioning Penn State for a unique focus on how we can develop autonomy that makes these operations safe. Johnson and Horn, for example, are working with Piasecki Aircraft Corporation to develop the autonomy for a manned helicopter that can automatically reconfigure in response to damage and faults, continuing their research into adaptive control. Likewise, Johnson is working with NASA to enable UAS to plan their trajectories to account not only for efficiency and mission goals, but also to keep in range of people-free zones so they can land immediately and safely in case of any problems.
Safety in Numbers
In a broader sense, our autonomy efforts are leading us to team with other domains, such as ground transportation, and with other disciplines, such as computer science, to lay down a fundamental science of safe autonomy.
We foresee several challenges. Safe autonomy will need to be interactive—we need humans and machines to interact fluidly and robustly with each other, as well as the vehicles to interact to create stable traffic flows. Safe autonomy will need to be adaptive, responding to new circumstances to maintain a safe state of operation. Autonomy serves to execute functions, or in a broader sense, operations, which can, themselves, be examined for their safety within a broader transportation system.
Finally, we need a broader viewpoint of how autonomy to date has evolved architectures for its hardware and software, incorporates sensors, makes judgments and decisions, and commands actions. These architectures have, in many cases, become overly complex, brittle to the unexpected, and fragile in the face of failures.
Thus, I envision our collective efforts now defining just valuable autonomous functions contributing to safety but also laying out broad systems perspectives on how autonomous vehicles should be architected from the start to be robust and resilient.
Amy Pritchett
Department Head
Professor of Aerospace Engineering
Department of Aerospace Engineering
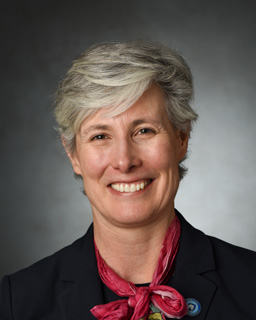
Amy Pritchett
Department Head
Professor of Aerospace Engineering
Department of Aerospace Engineering